Bruce McEwen is Alfred E Mirsky professor of neurosciences and behaviour and head of the Harold and Margaret Milliken Hatch Laboratory of Neuroendocrinology at Rockefeller University in New York City. His award-winning research on stress and the brain has been published in Proceedings to the National Academy of Sciences, Journal of Neuroscience, and Molecular Psychiatry. He lives in New York.
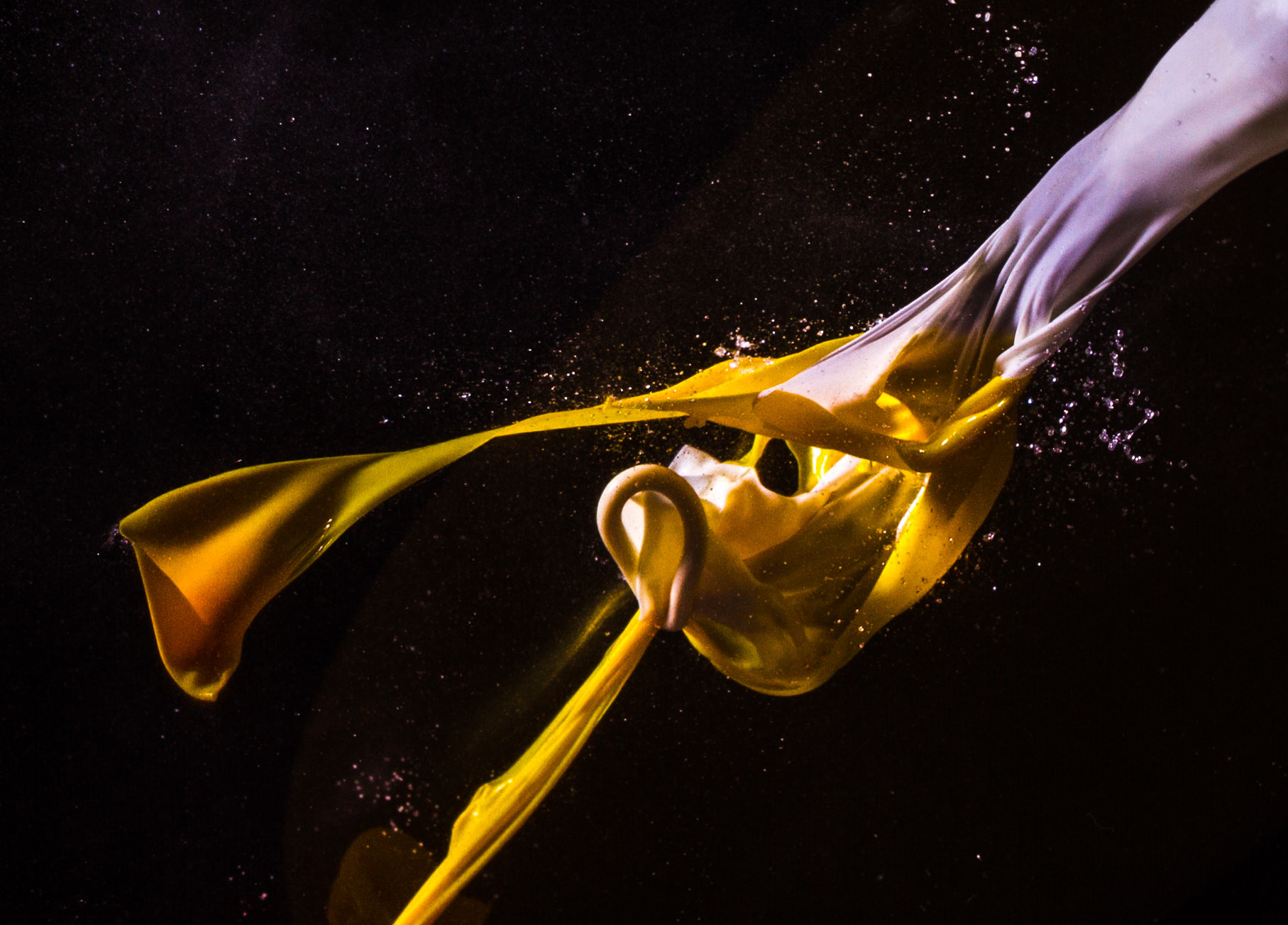
Breaking point. Photo by Paul Furborough/EyeEm/Getty Images.
Stress pervades our lives. We become anxious when we hear of violence, chaos or discord. And, in our relatively secure world, the pace of life and its demands often lead us to feel that there is too much to do in too little time. This disrupts our natural biological rhythms and encourages unhealthy behaviours, such as eating too much of the wrong things, neglecting exercise and missing out on sleep.
Racial and ethnic discrimination, along with lack of educational opportunities and economic advancement take their toll on a large segment of the population in the United States. Incarceration is the rule rather than the exception for some of the most vulnerable. Adverse experiences in infancy and childhood, including poverty, leave a lifelong imprint on the brain and body, and undermine long-term health, increasing the incidence of cardiovascular disease, diabetes, depression, substance abuse, anti-social behaviour and dementia. How does all of this stress ‘get under our skin’? What does it do to our brains and our bodies? What can we do about it? And is stress so multifaceted and pervasive that we could have trouble controlling it at all?
The psychologist Jerome Kagan at Harvard University recently complained that the word ‘stress’ has been used in so many ways as to be almost meaningless; he suggests it’s warranted only for the most extreme circumstances or damaging events. But my decades of experience suggest another approach. The insidious power of stress to ‘get under the skin’ was the focus of a MacArthur Foundation Research Network that I joined more than two decades ago, uniting me with social scientists, physicians and epidemiologists around a common problem: how to measure and evaluate stress from our social and physical environments. Our collaboration, continued under the auspices of the National Scientific Council on the Developing Child, has shown that stress acts on the body and brain, profoundly influencing health and disease.
Our findings are nuanced, starting with the fact that not all stress is the same. ‘Good stress’ involves taking a chance on something one wants, like interviewing for a job or school, or giving a talk before strangers, and feeling rewarded when successful. ‘Tolerable stress’ means that something bad happens, like losing a job or a loved one, but we have the personal resources and support systems to weather the storm. ‘Toxic stress’ is what Kagan refers to – something so bad that we don’t have the personal resources or support systems to navigate it, something that could plunge us into mental or physical ill health and throw us for a loop.
Now let us put these three forms of stress into a biological and behavioural context by invoking ‘homeostasis’ – the physiological state maintained by the body to keep us alive. It is through homeostasis that we maintain body temperature and pH (alkalinity and acidity) within a narrow range, keep our tissues perfused with oxygen and our cells fed. To maintain this steady state, our body secretes hormones such as adrenalin. Indeed, when we encounter an acute perceived threat – a large, menacing dog, for example – the hypothalamus, at the base of our brain, sets off an alarm system in our body, sending chemical signals to the pituitary gland. The pituitary, in turn, releases ACTH (Adrenocorticotropic hormone) that activates our adrenal glands, next to our kidneys, to release adrenalin and the primary stress hormone, cortisol. Adrenalin increases heart rate, blood pressure and energy supplies; cortisol increases glucose in the blood stream and has many beneficial effects on the immune system and brain, among other organs. In a fight-or-flight situation cortisol moderates immune-system responses, and suppresses the digestive system, the reproductive system and growth processes, as well as signalling brain regions that control cognitive function, mood, motivation and fear.
Biochemical mediators such as cortisol and adrenalin help us to adapt – as long as they are turned on in a balanced way when we need them, and then turned off again when the challenge is over. When that does not happen, these ‘hormones of stress’ can cause unhealthy changes in brain and body – for example, high or low blood pressure, or an accumulation of belly fat. When wear and tear on the body results from imbalance of the ‘mediators’, we use the term ‘allostatic load’. When wear and tear is strongest, we call it allostatic overload, and this is what occurs in toxic stress. An example is when bad health behaviours such as smoking, drinking and loneliness result in hypertension and belly fat, causing coronary artery blockade. In short, the mediators that help us to adapt and maintain our homeostasis to survive can also contribute to the well-known diseases of modern life.
***
The word stress is often explained as a ‘fight-or-flight response’. But what really affects our health and wellbeing are the more subtle, gradual and long-term influences from our social and physical environment – our family and neighbourhood, the demands of a job, shift work and jet lag, sleeping badly, living in an ugly, noisy and polluted environment, being lonely, not getting enough physical activity, eating too much of the wrong foods, smoking, drinking too much alcohol. All these contribute to allostatic load and overload through the same biological mediators that help us to adapt and stay alive.
Even though we now know all this, we often hear that measuring our cortisol levels will tell us if we are stressed. This reflects a misunderstanding at two levels. First, a single measure of cortisol will tell us nothing since cortisol levels go up and down within minutes – and halting this fluctuation impairs ongoing adaptive plasticity within the brain. Moreover, cortisol fluctuates throughout the day, going up in the morning to awaken us and then declining, except for a rise at lunch time, until it falls to low levels in the evening before we go to bed. Flattening this diurnal rhythm is a consequence of sleep deprivation and certain forms of major depression; a flat rhythm not only attenuates a robust, adaptive cortisol stress response but it also promotes obesity and high cholesterol, risk factors for diabetes and cardiovascular disease. It does so in part by causing the liver to make the ingredients to deposit body fat.
Cortisol is not the ‘bad guy’: it has a normal physiological role, coordinating the metabolism with activity and sleep
There are several ways to measure cortisol to determine whether the normal daily rhythm has been derailed. We can collect urine overnight or over the day. We can measure cortisol in hair from the forehead, which gives an index of our cortisol production over days. Or we can measure cortisol in our saliva at multiple times during the day, or before, during and after a stressful challenge, such as talking about something personal before a group of strangers. The stressful challenge gives us a picture of the efficiency of our allostasis – marked by turning up our cortisol response when challenged and needed for adaptation to maintain homeostasis, and then turning it off when the stressor is over so as not to produce adverse effects of allostatic load and overload.
Failure to turn on cortisol when needed is bad, leaving the door open for the body’s inflammatory response to compensate in an imperfect way. Too much inflammation can kill us as in septic shock. Failure to turn off cortisol after the stress is over produces negative effects too. Among the consequences are an increase of fat production, leading to obesity, diabetes, depression and eventual heart disease – all contributors to allostatic load.
Given our need for a robust cortisol response in the face of stress, the second misunderstanding about cortisol is the notion that it’s the ‘bad guy’. Rather, cortisol has a normal physiological role; it helps us adapt to stressors and coordinates our metabolism with daily activity and sleep patterns. We would not live very long or well without our cortisol! As my former student Firdaus Dhabhar, now a neuroimmunologist at the University of Miami, found, the early morning rise of cortisol, along with the stress response, activates immune function so that we can fight an infection or repair a wound. Likewise, the normal ‘morning awakening’ rise of cortisol that helps rouse us and makes us hungry for breakfast enhances the body’s response to immunisation if administered in the morning. The body’s response is like an orchestra involving many players working in harmony.
***
If the body functions like an orchestra, the conductor is the brain. It stores memories from bad as well as good experiences, and works with the body to keep us alive by minimising those subtle and long-term influences that cause allostatic load and overload. What we call the ‘wisdom of the body’ refers back to allostasis, the active process of biological adaptation and its role in maintaining homeostasis. Indeed, the brain is a plastic and vulnerable organ, continually sculpted by experience. It changes its architecture and function as part of allostasis. One recent study shows how the brain architecture of a mother is sculpted during pregnancy as part of the formation of attachment to the child. Other studies show how musicians’ brains develop, with greater skill leading to an increased neuron size and enhanced connections between sensory and motor-control regions of the cerebral cortex.
As opposed to motherhood and musicianship, toxic stress can increase anxiety by causing neurons in the amygdala, a brain region controlling anxiety and aggression, to become larger. Mindfulness practices such as meditation can reverse the process and reduce the size of those neurons, along with the stress. And regular physical activity, such as walking every day, causes genesis of new neurons in the hippocampus, a brain region that is essential for daily memory and spatial orientation; and it also improves memory and mood.
We also need to consider where our genes fit in, and understand that they do not rigidly determine our destiny, but rather provide the foundation on which our experiences shape our brains and bodies over the life course via ‘epigenetic’ mechanisms, which operate ‘above the genome’ – controlling the expression of genes without changing the genetic code. Epigenetics drives the seamless integration of experiences, both good and bad, acting on our genetic code over our life course. We now understand that epigenetics is the means by which stress acts on the body, the genome, and the brain.
My life’s work has helped me to tell the story of stress, starting with the mentors for my dissertation, completed in 1964. These two Rockefeller University scientists, Vincent Allfrey and Alfred Mirsky, taught me the fundamentals of epigenetics in the 1960s, before there was much interest in it, and when epigenetics meant something quite different, namely, the emergence of characteristics as a fertilised egg developed into a living organism. Development from embryo to independence is programmed into each species, but the individual characteristics that emerge are influenced by experience, and that is where the modern use of ‘epigenetics’ comes from. An example of this is a pair of identical twins with genes that predispose them to schizophrenia or bipolar illness. Even with the same DNA, the probability that one twin will develop the disease when the other twin gets it is only in the range of 30-60 per cent, which leaves plenty of room for experiences and other environmental factors to either prevent or precipitate the disorder.
Allfrey and Mirsky studied proteins called histones, which package and order DNA. Histones can be chemically modified to unwind the double helix, allowing genes to be expressed. Around 1960 , researchers showed that hormones such as cortisol and oestradiol used this mechanism to turn on genes in the uterus and liver, and this became a focus of my work in 1966.
In conditions of toxic stress, the same organs of the body are targeted for damage
Before long, I had changed my focus from the liver to the brain. As with cortisol in the liver, hormones of the adrenals and gonads could alter gene expression in the brain, working synergistically with other biochemical mediators to alter brain structure and function. Because experience itself affected these hormones, experience moulded what was now called the ‘epigenetic effects’.
This led to the finding that the adrenal stress hormone cortisol acts epigenetically on a brain structure called the hippocampus, which we now know mediates memory of daily events in space and time and also regulates mood. In other words, the hippocampus is a ‘GPS’ of the brain, a discovery that in 2014 saw the Nobel Prize awarded jointly to the UK-based neuroscientist John O’Keefe and the Norwegian scientists May-Britt Moser and Edvard Moser.
The hippocampus has since become a gateway into learning how sex hormones, metabolic hormones and stress hormones enter the brain, bind to receptors and act epigenetically to positively regulate structure and affect our behaviour. It has also helped us study conditions of toxic stress, when the same hormones and mediators contribute to allostatic overload; when that happens, the organs of the body, including the heart and the brain, are targeted for damage in a toxic storm.
Over the course of decades, my laboratory participated in, and in some cases initiated, these discoveries with the help of some remarkable students, postdoctoral fellows and colleagues. Among them was Ron de Kloet, now a professor at the University of Leiden, who studied the impact of synthetic glucocorticoids, which serve as potent inhibitors of inflammation and immune function, and stimulators of liver glucose metabolism (hence the name ‘glucocorticoid’). Cortisol is a natural glucocorticoid, and de Kloet found that synthetic glucocorticoids such as the medication dexamethasone (DEX) are actively excluded from the brain while cortisol gets in. But when a medication is given to quell inflammation, it can shut off the body’s ability to make cortisol. Then, when DEX treatment is terminated, the body and brain become deficient in cortisol, causing terrible mood swings and metabolic and immune disruption. After that, de Kloet went on to show, in his own laboratory with his student Hans Reul (now professor at the University of Bristol) that cortisol in the hippocampus binds to two receptor types, called MR and GR, to produce its myriad of important actions in the brain.
Another important advance was made by a student in my laboratory, Robert Sapolsky, now a professor at Stanford and a well-known author of a number of books, who found that, over the lifespan of a rat, the cortisol equivalent in the rat – corticosterone – gradually causes ‘wear and tear’ on the hippocampus, impairing not only memory and mood but also the ability to shut off the production of its glucocorticoids. This effect is more evident in animals and people who have experienced toxic stress. The ‘glucocorticoid-cascade hypothesis of stress and ageing’, as it is called, was the basis for the concept of allostatic load and overload. Sapolsky also did seminal work on dominant and subordinate baboons in Africa, and laid the groundwork for how income, education and human social hierarchies impact physical and mental health.
***
Until we grasped the impact of epigenetics, the brain was regarded as structurally stable in adult life, and the main focus for understanding normal and abnormal brain function was neurochemistry and neuropharmacology. During the 1980s, practitioners relied largely on antidepressants such as Prozac along with an array of antipsychotic medications to help patients heal.
Then in 1988, Elizabeth Gould, now a neuroscientist and professor at Princeton, came to my laboratory as a postdoctoral fellow. She introduced us to an old method hailing from the late 1800s and to Camillo Golgi, an Italian neuroanatomist who won a Nobel Prize for it. The Golgi technique, when done right, allows the investigator to visualise and measure the dendrites (like tree branches) emerging from neurons, and even the spines (sites of synapses, or connection, with other neurons) on those dendrites. Using the Golgi technique, Gould together with the Japanese biological psychiatrist Yoshifumi Watanabe showed that dendrites shrink and spine synapses are lost on hippocampal neurons after chronic stress lasting several weeks. The effect was due, in part, to the actions of glucocorticoids such as cortisol. In contrast, Catherine Woolley (now a professor at Northwestern University) showed that spine synapses come and go during the rat’s oestrous cycle (comparable to the human menstrual cycle) because of the fluctuations of the ovarian hormone oestradiol and progesterone.
Remarkably, in both cases, the hormones did not work alone and required, among other mediators, the main neurotransmitter in the brain, glutamate. Thus, circulating hormones not only enter the brain and bind to receptors but also participate with the brain’s own neurotransmitters in what we now call ‘adaptive plasticity’ – structural changes in the brain to enhance our success and survival. Adaptive plasticity underlies behavioural and neurological adaptation to the world. For example, shrinkage of dendrites in the hippocampus protects those neurons from damage by over-stimulation during toxic stress. Cyclic fluctuations of spine synapses during the oestrous (and human menstrual) cycle underlie differences in behaviour, including mood swings. The actions of oestradiol on cognitive function and their absence after the menopause have become the focus of hormone therapy to slow cognitive ageing and prevent Alzheimer’s disease, and the work on this topic by my colleague John Morrison, now director of the Primate Research Center at University of California, Davis (some in collaboration with us) has been very influential. Likewise, the contributions by our former postdoctoral fellow Roberta Brinton, now professor at the University of Arizona, have opened new avenues for use of the hormone progesterone as a protective agent for the ageing and damaged brain.
Gould and her students Woolley and Heather Cameron (now principal investigator at the US National Institute of Mental Health) also established that neurons of the dentate gyrus, part of the hippocampus, die and are replaced via the process of neurogenesis, which continues over the entire life course. They found that toxic stress suppresses that neurogenesis, and shrinks the hippocampus, while other laboratories went on to show that physical activity increases neurogenesis not only in young but also in older animals.
Regular physical activity is the most important behaviour that one can do to maintain brain and body health
These revelations about adult brain neurogenesis have huge implications not only because of the recognition that stem, or progenitor, cells might be used to treat brain damage, but also because of their meaning for lifestyle. Regular physical activity increases this neurogenesis in old as well as young people, and improves memory and mood and even enlarges the hippocampus, which tends to shrink in depression and diabetes among other conditions. Within six months to a year, regular aerobic activity such as walking an hour a day five out of seven days a week not only makes the hippocampus larger and improves memory but also improves decision-making by improving blood flow and metabolic function in the prefrontal cortex, a brain region essential for self-regulation of emotions and impulses as well as working memory. Indeed, regular physical activity is the most important behaviour that one can do to maintain brain and body health. And, as a further illustration of brain-body communication, the ability of exercise to stimulate neurogenesis requires that at least two hormones be taken up from the body into the brain. One of them, IGF-1, comes from the liver, and the other, cathepsin B, comes from muscle.
Plasticity of the brain extends to the diurnal cycle of waking and sleeping, and reaches beyond the hippocampus to other brain regions. A former student, Conor Liston, now an assistant professor of psychiatry at Weill Cornell Medical School, found that some, but not all, synapses in many parts of the cerebral cortex turn over during the day-night cycle due to the fluctuation of cortisol. Interfering with that cycle at the wrong time of day interferes with motor learning, eg learning to play golf. Considering how many ways we modern humans interfere with our natural day-night rhythm – for example, by turning on a light in the middle of the night – this is a lesson to all of us to give the ‘wisdom of the body’ a better chance to help us.
Another way that we interfere with the natural cycle is through shift work and jet lag. Our former post-doctoral fellow Ilia Karatsoreos, now an associate professor at Washington State University, found that creating an animal model of shift work caused dendrites in the prefrontal cortex (the brain region that governs our ability to regulate emotions and impulses, as well as working memory) to shrink and the animal to become cognitively rigid when challenged with a memory task that required changing the rules. Moreover, the shift-work animals became fatter and insulin-resistant, signs of pre-diabetes and depressive-like behaviour. Shift work in our own species is associated with greater obesity, diabetes, cardiovascular disease and mental-health problems.
The prefrontal cortex also responds to what we can call ‘tolerable stress’. During his MD-PhD thesis research, Liston assessed a group of medical students for perceived stress (how much or little they felt in control of their lives). He found that those with the highest perceived stress were slower in doing a cognitive-flexibility test, and also had slower functional connectivity in a brain circuit involving the prefrontal cortex when tested in a fMRI machine. The reason we can call this ‘tolerable stress’ is that, after a vacation, these impairments disappeared, showing the resilience of the young adult brain. Parallel studies of perceived stress on an animal model allowed Liston to see shrinkage of neuronal dendrites and reduction of synapses in the prefrontal cortex that explained the deficits in cognitive flexibility.
***
To complete the story of brain plasticity we need to describe how the same stressors cause dendrites to shrink and synapses to be lost in the prefrontal cortex and hippocampus. The answer comes from Sumantra Chattarji, a professor at India’s National Centre for Biological Sciences in Bangalore, and his team: dendrites in the basolateral amygdala, the seat of fear and anxiety and strong emotions, grow and become more branched, increasing a sensation of anxiety.
Liston found that dendrites in the orbitofrontal part of the prefrontal cortex also expand, increasing vigilance. In the short term, these changes might be adaptive, because anxiety and vigilance can aid us during dangerous or uncertain times. But if the threat passes and the behavioural state ‘gets stuck’ and persists along with changes in neural circuitry, such maladaptation requires intervention to open ‘windows of plasticity’ with a combination of pharmacological and behavioural therapies.
Again, regular physical activity can strengthen both prefrontal cortex and hippocampus control of the amygdala. This means that we are better able to control moods and emotions as well as impulses, and are more efficient in making decisions. Another approach to chronic anxiety is mindfulness-based stress reduction (MBSR), which has been shown to decrease the amygdala in some. Both MBSR and meditation are gaining in popularity as a way of reducing anxiety and thus reducing perceived stress.
Some of this research also has implications for post-traumatic stress disorder (PTSD). Chattarji has found that a single, traumatic stressor can lead to formation of new synapses in the basolateral amygdala after a week or two. The appearance of those new synapses is accompanied by a gradual increase in anxiety. This type of delay is a feature of PTSD. What we have shown with Chattarji is that a timed elevation of cortisol at, or shortly after, a traumatic stressor actually prevents the delayed increase in amygdala synapses. Now there is evidence that low cortisol at the time of trauma – during open-heart surgery or after a traffic accident – is a risk factor, and that rising cortisol during or right after trauma can reduce later PTSD symptoms.
Also, our (and other) research shows that the impact of toxic stress varies across sex. Women are more prone to depression after toxic stress, while men are more likely to respond with antisocial behaviours and acts. We and others find that there are receptors for both oestrogens, androgens and progestins in both the male and female brain that regulate memory, pain, coordinated movement and other critical functions. But thanks to genetically programmed sex differences in our brains, men and women respond differently to stress. These sex differences occur throughout the brain and not just in regions such as the hypothalamus that are involved in reproduction. In fact, new research indicates that, at the molecular and genetic level, male and female responses to stress in the hippocampus can be strikingly different. Sure, studies of men and women’s brain activity show that both sexes do many of the same things equally well, but use somewhat different brain circuits to do so – lending some credence to the ‘men are from Mars, women are from Venus’ hypothesis!
Stress hits us differently depending on our experience early in life. Our former postdoctoral fellow Michael Meaney, now professor at McGill University, has led the way in demonstrating the important role of postnatal maternal care in emotional and cognitive development. Infant rats raised with a nurturing mother develop less emotionality and greater ability to explore novel places and things. Pups raised with an anxious mother who provides inconsistent care show the opposite outcome.
Epigenetics also plays a role. This is clear from studies looking at cross-fostering of infants between good and bad mothers. Switching mothers and pups alters the outcome, pointing to what is now referred to as epigenetic behavioural transmission.
Complementing this, we now know that, even before conception and during life in the womb, paternal and maternal obesity can affect the child. This might involve ‘epigenetic’ changes of the DNA of the sperm and egg that do not alter the genetic code per se, but, rather, how it is read; parental obesity increases the risk that the child will also become obese. Women who lose weight through gastric bypass surgery prior to conception do not transmit obesity to children, while those who remain obese through gestation put children at higher risk.
We can never reverse the effects of experiences, positive or negative, but we can move on to recovery and redirection
Adverse early life experience involving poverty, abuse and neglect affects how genes are expressed, and determines how well brain regions such as the hippocampus, amygdala and prefrontal cortex develop and function during childhood into young adulthood. Indeed, the brain is continually changing with experience, which creates memories and alters brain architecture via mechanisms that are facilitated in part by circulating sex, stress and metabolic hormones and chemicals produced by the immune system.
These insights have led to a new view of epigenetic changes over the life course. Epigenetic changes determine trajectories of health and disease and the plasticity of the brain. But they also offer opportunities for changing the trajectory as life goes on.
We can never roll back the clock and reverse the effects of experiences, positive or negative, or the epigenetic change they produce. But we can move through those experiences to recovery and redirection; also, we can develop resilience through epigenetic change. New trajectories can engender compensatory changes in the brain and body over the life course.
This perspective has led to a new field of study, called ‘life course health development’ (LCHD), spearheaded by Neal Halfon, a researcher and paediatrician at the University of California, Los Angeles. LCHD emphasises the importance of events prior to conception and in the womb because of their ability to generate epigenetic change; for the same reason, LCHD looks to the influence of income, education and abuse.
In synch with this, our increasing knowledge of brain plasticity is giving rise to therapies based on self-regulation. These cognitive techniques, tapping mindfulness, breathing and more, can reduce toxic stress to at least more tolerable stress. Metabolic and cardiovascular health, not to mention memory and mood, can all be enhanced by a healthy diet, positive social interactions, adequate sleep, and regular physical activity. Government policies and business cultures that promote these values are key – whether dealing with housing, transportation, healthcare, education, flexible working hours or vacations, decisions at the top can dramatically impact healthspan of the population throughout life. Healthy behaviours and humanistic policies can ‘open a window’ of plasticity and allow the wisdom of the body to exert itself. With the windows open, targeted behavioural interventions – for instance, intensive physical therapy for stroke – can shape brain circuits in a more positive direction. Even if one has gotten off to a bad start in life, the trajectory can be changed by understanding how to lower the allostatic load and banish toxic stress.