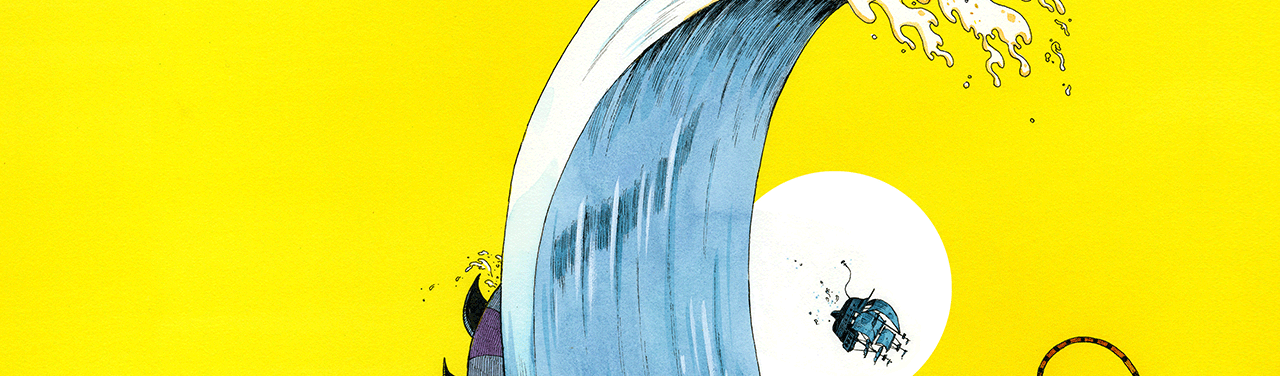
Illustration by John Hendrix.
Early in the morning on Sept. 11, 1995, the cruise liner the Queen Elizabeth 2, on its way from Southampton to New York, was being lashed by the tail end of Hurricane Luis, somewhere off the coast of Newfoundland. As if sensing its imminent demise, Luis had galvanized one last time, twitching to life and whipping the North Atlantic into a torrent of 130 mph winds and 40-foot waves. None of this caused undue concern for the ship’s captain, Ronald Warwick, a 30-year sailing veteran well acquainted with rough seas. Luis was hardly unexpected; since leaving England, the ship had steadily tracked the storm’s path. “This was fair game for us,” the retired Commodore recalls, from his home in Somerset, England. “We are a transatlantic liner.”
At dinner, Warwick had advised the ship’s passengers that things might get a bit rough during the night, and to secure any loose possessions in their cabin. He then did what any captain would do in heavy seas. With the ship steered into the waves, Warwick slowed it to a few knots. “Heaving to” it is called, the idea being to ride out the waves as gently as possible, while maintaining just enough speed to maintain steering control. Far below the ship’s bridge, where winds roared and whipped against the armored windows, a handful of passengers held casual vigil in a bar.
The anomalousness of this one wave still haunts Warwick.
Just after 2 a.m., Warwick and his officers suddenly saw a surging monster of water and convulsive white froth in the near distance. “If you’re standing on the bridge of the QE2,” he says, “your height of eye above sea level is 90 feet.” From what they could discern through the rain-soaked darkness, the crest of the looming wave was as high as the bridge itself. Warwick, who says he had never encountered anything so large in the ocean before, said it was as if they were heading into the “white cliffs of Dover.”
A minute or so later, the wave crashed across the forward deck of the QE2. “We didn’t go over the top of it,” says Warwick. “We virtually went through it.” A series of judders shot through the ship. The wall of water crashed down on the ship’s deck, buckling its steel plates some 18 inches. Still, this was all within the operating bounds of the ship. “We weren’t knocked off our feet or anything,” he says. “Most passengers were in bed.”
But the anomalousness of this one wave still haunts Warwick. For an old North Sea hand, 40-foot waves, the kind that would terrify most of us, were nothing out of the ordinary. But the emergence from nowhere of a single wave that was more than twice as high as the others was exceptional. Warwick had encountered a rogue wave.
In the common parlance, “rogue wave” has come to mean any wave that is unusually large. But a rogue wave does not have to be big on an absolute scale, nor is it necessarily associated with a heavy storm. Burkard Baschek, director of the Institute of Coastal Research, in Geesthacht, Germany, defines it as any wave that is statistically extreme. “[A rogue wave is] at least twice as high as the so-called significant wave height,” he says. The significant wave height is defined as the average of the largest one-third of waves at any given moment, “the waves you would see by eye if you’re out there and looking at the sea state.”
This means you have probably experienced a rogue wave and not noticed. How? “If you’re out in calm conditions,” says Baschek, “and the waves are one meter high, and suddenly you experience a two meter wave, so what?” In fact, Baschek says one wave per day, somewhere in the world’s oceans, is a rogue wave. While at the University of California, Los Angeles (UCLA), he analyzed data from a number of buoys off the west coast of the United States and, extrapolating the figures globally, estimated that ships trawling the North Atlantic (like the QE2) would “encounter 20-30 of these rogue waves during their service lives of 25 years,” or about one a year.
That rogue waves are, at base, mathematical objects has meant that sailors, then scientists, have been slow to grasp their nature. Late into the 19th century, the line between sailors’ lore and science could be as blurred as a fog-bound horizon, with reputable journals routinely reporting on the sightings of “monsters of the sea.” By the mid-20th century, though, most of these monsters had been dispelled or explained—but not rogue waves, which continue to be misidentified and incompletely understood today. Even one of civilization’s most famous waves, Hokusai’s The Great Wave off Kanagawa , is often misinterpreted as a tsunami—the woodcut has even been used as the symbol of a UNESCO tsunami warning sign—when it is actually more likely a rogue wave, as several scholars have argued. 1,2

Surf’s up: “The Great Wave off Kanagawa” by Hokusai is often assumed to depict a tsunami, but is more likely to be a rogue wave. Photo from Wikipedia.
The science of rogue waves began to take shape in the early 1960s, not in the ocean but in a wave tank—essentially a long, shallow trough with a “paddle” for generating waves of varying frequencies—in Cambridge University’s Engineering Department. There, the physicist and fluid dynamics expert T. Brooke Benjamin, aided by a graduate student named Jim Feir, were having trouble trying to prove something that had long been assumed: the stability of the so-called “Stokes wave,” the sort of Ur-wave, or prototypical wave, of fluid dynamics, theorized by the renowned 19th-century Cambridge University mathematician George Gabriel Stokes. Stokes, inspired by a British engineer’s faintly astonished report of a “solitary heap of water,” pushed along by a slowing boat, that had maintained its speed and shape for nearly two miles as it traveled through a canal, looked for an equation that could describe a wave propagating in shallow water without changing shape. 3
Rough seas A toy boat rides over waves created by a paddle in a Hamburg wave tank. Small perturbations to the behavior of the paddle grow “due to the natural instabilities of the water waves themselves,” says physicist Nail Akhmediev. This occasionally produces rogue waves like the one that topples the boat. Akhmediev says he has seen rogue waves as large as five times the significant wave height. Credit: YouTube / SteveHOCP.
The effect, now known as the “Benjamin-Feir instability,” offered a hint as to how rogue waves form. As J.B. Zirker describes in his book, The Science of Ocean Waves , the wave group, once it found a kind of sweet spot of modulation, stole energy from itself to produce a single high wave—higher than might be expected from the normal dynamics of the waves—then “returned” it to the primary wave. “The sidebands grew exponentially in height at the expense of the primary wave, which eventually disintegrated,” he writes. “Nobody had ever seen or expected the likes of this before.”
What ultimately made the concept of rogue waves so striking was not simply that they could grow to unexpected heights, but that they occurred more frequently than they were expected to. As Eric Heller, a physicist at Harvard University, explains it, much of the thinking on large wave creation was influenced by M.S. Longuet-Higgins, the noted mathematician and oceanographer, who was, as he wrote in one study, trying to “study theoretically the statistical properties of a random, moving Gaussian surface.” In other words, the ocean.
“His theory was based on the random addition of waves,” Heller says. “He was uniformly adding them up over large areas and asking how many unlucky additions you would get resulting in large waves.” It was a linear process. According to Heller, there was just one problem: “There were more freak wave events [in reality] than that theory could ever really account for. Light bulbs went off in people’s heads—it must be nonlinear evolution!”
And so rogue waves crashed into the longstanding “Gaussian seas” model of randomly distributed wave heights. They were not quite as long-tail as had been thought, and new probabilities, like the so-called “Tayfun distribution” (which coincidentally sounds like “typhoon” but is named for a Turkish scholar) have gained currency to predict the likelihood of rogue waves.
One of the biggest boons to the study of rogue waves came not in the laboratory, but in the ocean itself. On the night in 1995 that the QE2 was struck by the giant wave, there was another witness, one who experienced the wave from a deeply immersive position: “Buoy 44139,” an instrumented floating beacon monitored by the Canadian Forces Meteorological Office at Halifax. The statistical graph it generated during the torment is simple, yet chilling. There is a slightly jagged saw-tooth line of wave heights, the 40-foot rollers that Warwick had hove to against. But then, with no anticipatory buildup, there rises a single, dramatic peak, leaping toward the top of the page, almost, literally, “off the charts.” Just as suddenly, it plunges again, as if it were never there.
Buoys like 44139, equipped with accelerometers and other measuring devices, brought precision to an arena where sailor’s accounts and estimations had dominated. Before their advent, gauging the heights of waves was an inexact process. One early, and complicated, effort involved photographing the ocean from an airplane and mathematically estimating wave height via the reflection of light. 6
But buoys are far from perfect. They get overtopped by waves, or knocked on their sides. There is also an issue of selection bias. Most instrumented buoys are located close to shore, says Baschek, while the largest rogue waves occur more frequently on the open ocean. Not all buoys can measure the direction of waves. And the big wave measurements that have been captured—the QE2, or, in another well-known instance earlier that same year, a 30-meter wave captured on the oil platform Draupner off the coast of Norway—are single point measurements. “It’s a buoy or a radar point,” he says. “But you have no idea what the crest length is. If you measure a rogue wave, is it one local spray, or is it going over miles and miles? How long does it persist? Does this rogue wave form right before your buoy and fall apart right after that? This is also something you don’t know.”
One of civilization’s most famous waves is often misinterpreted as a tsunami.
As a striking reminder of how science, even with its ever more sophisticated and powerful measurement capabilities, is still catching up with the enormity of the ocean, it was only in 2011 that the long-suspected, but never observed, phenomena of “merging tsunami”—two waves (formed non-linearly) becoming (linearly) a more powerful one—was finally observed in the wild, thanks to a procession of satellites orbiting over the tsunami. The capture of the merging tsunami was a “one in 10 million chance” random observation, as an investigator put it. 7
For these reasons, even in this age of networked data and supercomputer simulations, some of the best data on rogue waves continues to come from the men and women who work on the sea.

In January, 2010, the crab boat Early Dawn (featured, as it happens, in the Deadliest Catch reality series), operating near the town of St. Paul, Alaska, was struck by a wave that was said to be twice as high as the significant wave height. In the course of a subsequent insurance investigation concerning injuries to a crew member, Baschek was called in to help answer a necessary but difficult question: Should the captain have known about the possibility of such a wave, and was he prepared enough? This involved the usual problem of the probabilities of such waves, with an unusual twist. The wave that struck the boat that winter did not come from the prevailing wave direction.
This violated the traditional linear theories, Baschek says, of the superposition of waves coming out of the same direction and combining into a larger wave. “This was a total puzzle,” he says. “Because we don’t know the physics behind it, all we could do was dig out the records we had of the West Coast of the U.S., about 100 years of total data.” The question they wanted to answer: How likely is it the rogue wave occurs at an angle that differs from the prevailing wave direction? He says they found a likelihood of about 1 percent. “It is now up to a judge to decide if this was likely or not,” he says. “That’s where science ends.”
As you travel down the tail of the probability distribution, rogue waves become ever stranger. Not only can their size and direction be unexpected, but they can appear in some unlikely places. When I reach Nail Akhmediev, a professor in the Research School of Physics and Engineering at the Australian National University in Canberra, and one of the world’s leading experts in nonlinear dynamics (he has a special kind of nonlinear wave, an “Akhmediev breather” to his name), he points out that our very conversation, conducted via Skype, may be subject to rogue waves.
“Right now, when we are talking, all this sound, it travels through optical fiber,” he says. “It’s waves. Whether ocean waves or waves in optical fibers, it’s basically the same equations.” In optical fiber, he notes, waves can be chaotic and turbulent. The pipes that connect Canberra and New York City are comprised of many channels—thousands of people talking at once. “In a fiber, it’s all unidirectional,” he says. “But because there are several channels, they cross each other.”
Under the right conditions, even rogue waves can appear, a fact first reported by UCLA physicist Daniel Solli and colleagues in a 2007 paper in Nature , in which they noted the appearance of “a noise-sensitive, non-linear process in which extremely broadband radiation is generated from a narrowband input.” And while it is unlikely that a particularly voluble Skype conversation is going to trigger a catastrophic pulse across global telecommunications networks, as physicist Neil Broderick points out, the emergence of optical rogue waves remind us that the nonlinear math looks much the same across waves in many different kinds of systems; perhaps “seed pulses,” he notes, could be used, along with artificial reefs, to create “perfect waves” for surfers. 8
This thought came to me as I watched a video of an experiment that Akhmediev and his colleagues conducted in a wave tank in Hamburg. In the video, a small Lego pirate ship sits gently bobbing atop the silky undulations of the tank’s mirror-surface water, as, unseen, a wave generator beats out a steady pulse of fluid dynamics. And then there it is, a dark band in the distance, which engulfs and capsizes the hapless boat. It is a “super” rogue wave, not twice the significant wave height, but five times. I envisioned, for a moment, an indoor water park where swimmers, placidly bathing, would be delightedly and unexpectedly jostled by exquisitely derived nonlinearity.
But the experiment was a stark reminder of how much mystery still lay in the generation of rogue waves. They are the ocean’s black swans. We know that rogue waves occur, in a general statistical sense, but we do not know where or when—or, fully, how—they will, or will not, occur. Perhaps there are even 5x rogue waves yet to be discovered in the world’s seas. It is not surprising, says Heller, that so much of wave science is about probability; even if we had all the data to understand the ocean, we would not have computers powerful enough to crunch it. “Statistics is very much a part of physics these days,” he says. “There will be cases in quantum mechanics where there’s nothing but statistics,” no way other than guesses as to where or even if a particle’s going to appear. Even the calmest sea is a veneer—an alibi—for chaos, actual and statistical.
Tom Vanderbilt writes on design, technology, science, and culture, among other subjects.
References
- Cartwright, J.H.E. & Nakamura, N. What Kind of a Wave is Hokusai’s Great Wave Off Kanagawa . Notes and Records: The Royal Society Journal of the History of Science 63 , 119–135 (2009).
- Dudley, J.M., Sarano, V., & Dias, F. “On Hokusai’s Great Wave Off Kanagawa : Localization, linearity and a rogue wave in sub-Antarctic Waters. Notes and Records: The Royal Society Journal of the History of Science 67 , 159-164 (2013).
- Zirker, J.B. The Science of Ocean Waves Johns Hopkins University Press (2013).
- Drazin, P.G. & Reid, W.H. Hydrodynamic Stability Cambridge University Press (2004).
- Hunt, J.C.R. Nonlinear and Wave Theory Contributions of T. Brooke Benjamin (1929-1995) Annual Review of Fluid Mechanics 38 , 1-25 (2006).
- Jahne, B., Schmidt, M., & Rocholz, R. Combined optical slope/height measurements of short wind waves: principle and calibration. Measurement Science and Technology 16 , 1937-1944 (2005).
- Buis, A. & Cole, S. NASA finds Japan tsunami waves merged, doubling power. NASA.gov (2011).
- Broderick, N. Viewpoint: Optical rogue waves on demand. Physics 3 , 101 (2010).