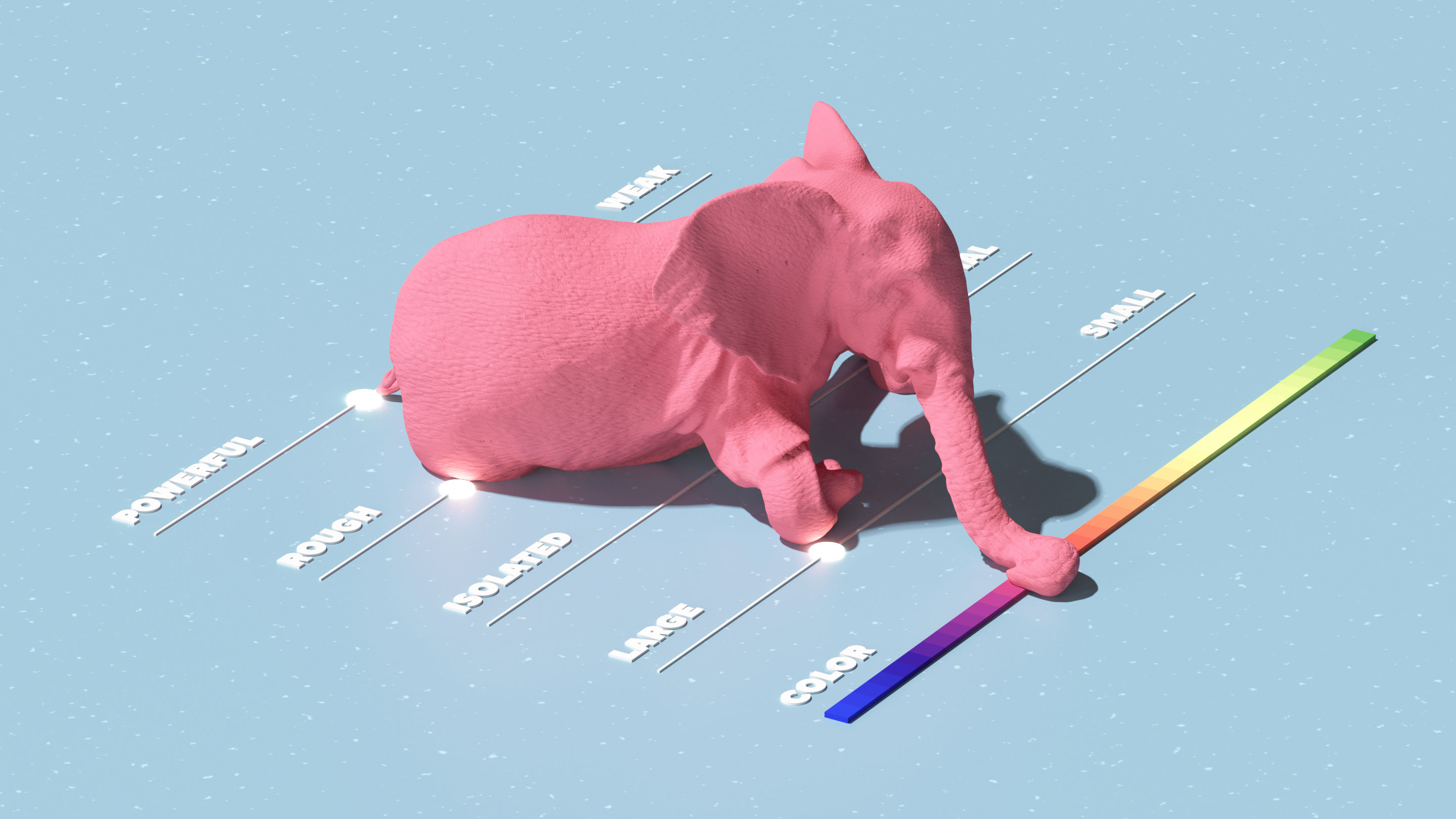
In the parable of the blind men and the elephant, each paid attention to a different aspect of the creature. The brain may do something similar by mapping out the qualities of perceptions, experiences and abstract concepts along various dimensions, with the help of the same system that it uses to map out physical spaces. Credit: Alexandre Tamisier for Quanta Magazine.
We humans have always experienced an odd — and oddly deep — connection between the mental worlds and physical worlds we inhabit, especially when it comes to memory. We’re good at remembering landmarks and settings, and if we give our memories a location for context, hanging on to them becomes easier. To remember long speeches, ancient Greek and Roman orators imagined wandering through “memory palaces” full of reminders. Modern memory contest champions still use that technique to “place” long lists of numbers, names and other pieces of information.
As the philosopher Immanuel Kant put it, the concept of space serves as the organizing principle by which we perceive and interpret the world, even in abstract ways. “Our language is riddled with spatial metaphors for reasoning, and for memory in general,” said Kim Stachenfeld, a neuroscientist at the British artificial intelligence company DeepMind.
In the past few decades, research has shown that for at least two of our faculties, memory and navigation, those metaphors may have a physical basis in the brain. A small seahorse-shaped structure, the hippocampus, is essential to both those functions, and evidence has started to suggest that the same coding scheme — a grid-based form of representation — may underlie them. Recent insights have prompted some researchers to propose that this same coding scheme can help us navigate other kinds of information, including sights, sounds and abstract concepts. The most ambitious suggestions even venture that these grid codes could be the key to understanding how the brain processes all details of general knowledge, perception and memory.
The Amnesiac and the Hexagons
On September 1, 1953, Henry Molaison, a 27-year-old man the world would come to know as “Patient H.M.,” went under the knife in a risky, experimental bid to cure a debilitating case of epilepsy. A neurosurgeon removed the hippocampus and surrounding tissues from deep within H.M.’s brain, alleviating some of his seizures but inadvertently leaving him a permanent amnesiac. Until his death more than half a century later, H.M. couldn’t encode new memories: not what he’d had for breakfast, nor the most recent news headline, nor the identity of the stranger he’d been introduced to just a few minutes earlier.
H.M.’s story, though tragic, revolutionized scientists’ understanding of the role the hippocampus plays in how the brain organizes memory.
Years later, another hippocampus-centered revolution transpired and earned its pioneers a Nobel Prize: the discoveries, decades apart, of two types of cells, which made it clear that the hippocampal region’s fundamental functions included not just memory but also navigation and the representation of two-dimensional spaces.
The first of these came in 1971, when researchers uncovered “place cells,” which essentially fire to indicate one’s current location. John O’Keefe, a neuroscientist at University College London, and his colleagues monitored the brain activity of freely roaming rats and observed that some of their neurons fired only when they were in specific parts of their cages. Some became active as a rat sniffed around, say, its enclosure’s northeast corner, but otherwise remained quiet; others fired in the cage’s center. That is, the cells encoded a sense of place (“you are here”) — and together, they created a map of the entire space. (When the rat was put in a different cage or room, these place cells “remapped,” encoding different local positions.)
These findings inspired the proposal that the hippocampus might be creating and storing “cognitive maps” (an idea first put forth by psychologist Edward Tolman in the 1940s to explain how rats could suss out new shortcuts to rewards in mazes) beyond spatial ones. At the very least, the hippocampus seemed like a promising place to start looking for hints of such maps.
That work eventually led a then-married pair of scientists at the Norwegian University of Science and Technology, May-Britt Moser and Edvard Moser, to direct their attention to the entorhinal cortex, located just next door to the hippocampus. The region provides major inputs to the hippocampus — and is also one of the first areas of the brain to deteriorate in Alzheimer’s disease, which affects both navigation and memory. There, the researchers found what they called grid cells, which experts now think may be the most compelling candidate for cognitive mapmaker.
Unlike the place cells, grid cells do not represent particular locations. Instead, they form a coordinate system that’s independent of location. (As a result, they’re popularly known as the brain’s GPS.) Each grid cell fires at regularly spaced positions, which form a hexagonal pattern. Imagine the floor of your bedroom is tiled with regular hexagons, all the same size, and each hexagon is divided into six equilateral triangles. As you walk across the room, one of your grid cells fires every time you reach a vertex of any of those triangles.
Different sets of grid cells form different grids: grids with larger or smaller hexagons, grids oriented in other directions, grids offset from one another. Together, the grid cells map every spatial position in an environment, and any particular location is represented by a unique combination of grid cells’ firing patterns. The single point where various grids overlap tells the brain where the body must be.
This kind of grid network, or code, constructs a more intrinsic sense of space than the place cells do. While place cells provide a good means of navigating where there are landmarks and other meaningful locations to provide spatial information, grid cells provide a good means of navigating in the absence of such external cues. In fact, researchers think that grid cells are responsible for what’s known as path integration, the process by which a person can keep track of where she is in space — how far she has traveled from some starting point, and in which direction — while, say, blindfolded.
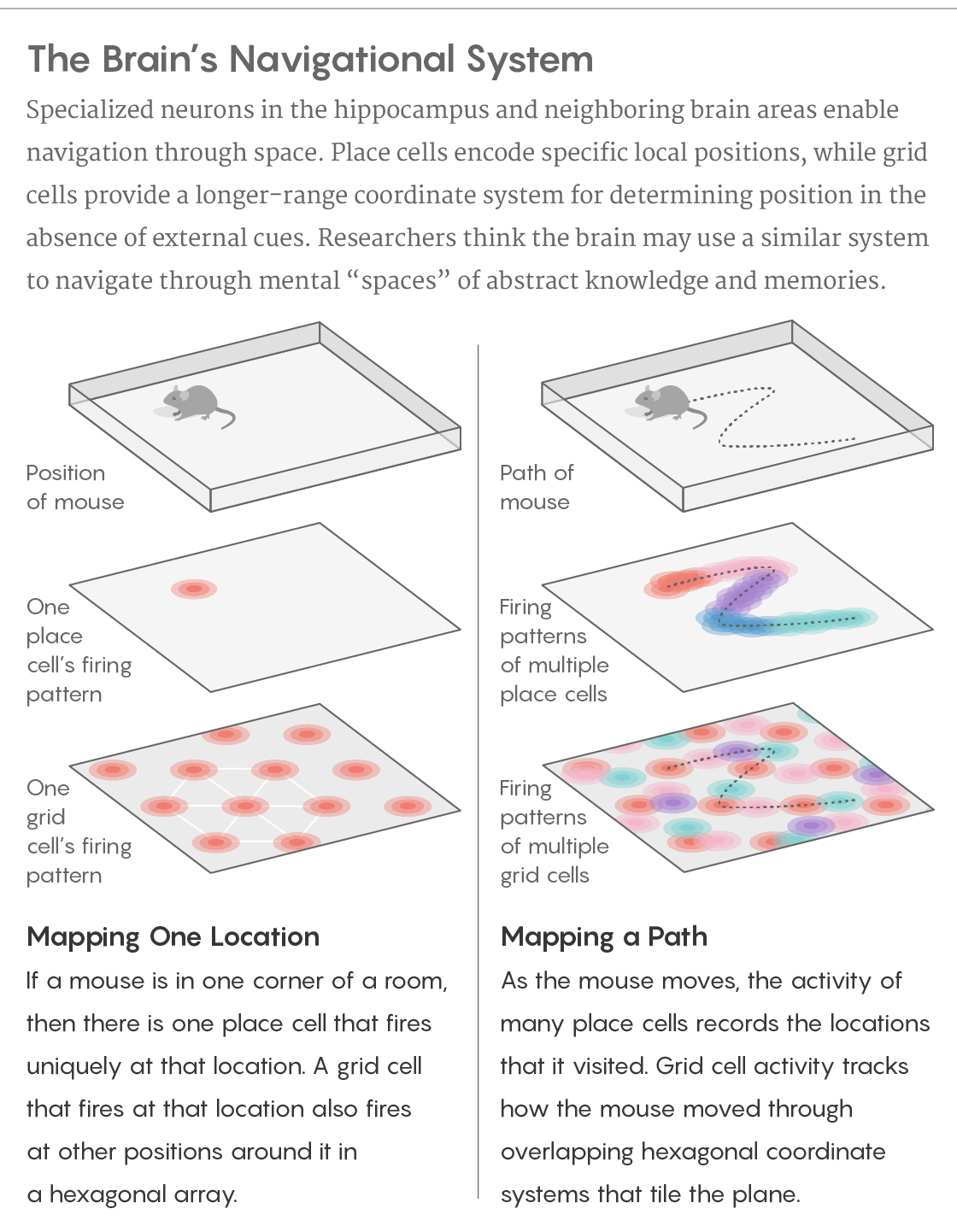
Credit: Lucy Reading-Ikkanda/Quanta Magazine.
“The idea is that the grid code could therefore be some sort of metric or coordinate system,” said Jacob Bellmund, a cognitive neuroscientist affiliated with the Max Planck Institute in Leipzig and the Kavli Institute for Systems Neuroscience in Norway. “You can basically measure distances with this kind of code.” Moreover, because of how it works, that coding scheme can uniquely and efficiently represent a lot of information.
And not just that: Since the grid network is based on relative relations, it could, at least in theory, represent not only a lot of information but a lot of different types of information, too. “What the grid cell captures is the dynamic instantiation of the most stable solution of physics,” said György Buzsáki, a neuroscientist at New York University’s School of Medicine: “the hexagon.” Perhaps nature arrived at just such a solution to enable the brain to represent, using grid cells, any structured relationship, from maps of word meanings to maps of future plans.
An Expanding Role for Grid Cells
“We’ve been thinking about how the hippocampus and entorhinal cortex machinery could have a more general purpose,” Stachenfeld said. “It’s a really powerful idea, that you can have a [grid cell] representation of structure in general, and apply it more rapidly to new situations.” That, in turn, would allow one “to behave more efficiently, to learn a lot faster.”
Since researchers usually could not take direct measurements of individual neurons in their test subjects, they had to get clever with their methodology. In 2010, for instance, neuroscientists figured out a certain kind of signal to look for in functional magnetic resonance imaging (fMRI) scans of the brain as an indirect signature of grid cell activity. This “hexadirectional” signal emerges in subjects navigating a virtual environment. As it turns out, it also characterizes other tasks, some spatial, some not so much.
One of the earliest examples came with behavior that fell somewhere between the two: the navigation of visual space. When monkeys, with their heads fixed in place, tracked images with just their eyes, researchers found evidence of grid cell activity in the entorhinal cortex. More recent work in humans has uncovered the same hexadirectional signature, and some experiments have even pinpointed other, more direct properties of the grid code already observed in physical navigation tasks.
Similar principles may also guide how the brain encodes time. The hippocampus has already been found to contain place cells that also behave as “time cell” neurons in certain situations, activating to indicate successive moments in time (rather than successive positions in space). Rats would run through a maze, in which one section involved trotting in place on a wheel or treadmill for some predetermined number of seconds before continuing onwards. During the interval when the rats ran in place, their actual location held constant, cells fired in their hippocampus to track their temporal progression: Some neurons were active for the first few seconds, others for the next few and so on. The finding “brings time as a different dimension into the equation,” Bellmund said.
More recently, work published in Nature last summer turned up evidence for a coding system that uniquely represents time in the context of memories or experiences. A team of researchers, led by the Mosers, uncovered a coding scheme for time that spanned multiple scales, from seconds to hours. Although no explicit link has yet been drawn between temporal organization and grid cells, scientists have seen hints of a connection: Grid cells signal elapsed time in rats running on treadmills, for instance.
Last year, a team of scientists at Princeton University brought yet another potential dimension into the mix: sound. They monitored brain activity in rats that were pushing a lever to change the frequency of an emitted tone to match one they had previously heard. Their observations hinted that the rats might be mentally navigating through an “acoustic space” in their minds to find the desired tone. [Editor’s note: This work was funded in part by the Simons Foundation, which also funds this editorially independent magazine.]
Perhaps most tantalizing of all, an experiment conducted in 2016 introduced a far more abstract context for grid cell behavior. Researchers led by Timothy Behrens, a computational neuroscientist at the University of Oxford, had people watch the silhouette of a bird on a screen as the length of its neck, the length of its legs or both were stretched and compressed. The hexadirectional signal arose in their fMRI data, in several areas of the brain; it varied just as if the test subjects were navigating a two-dimensional “bird space,” where one axis denoted neck length, the other leg length.
The finding suggested that the brain processes trajectories through physical spaces and conceptual spaces in much the same way. Now, researchers including Behrens, Bellmund and neuroscientist Christian Doeller propose that all knowledge can be plotted this way, in terms of features of interest — that different objects, different experiences and different memories can be organized and traversed with the grid code.
“It seems to be quite arbitrary, what dimensions it can map,” Bellmund said. “What’s interesting is that it seems to be so general across domains, but the mechanism seems to be preserved.”
This work, added Thomas Wolbers, a cognitive neuroscientist at the German Center for Neurodegenerative Diseases, calls into question the idea that grid cells simply constitute “a pure location signal” — hardwired and specialized. “So far, we’d only seen it in space because we’d only looked at navigation tasks and paradigms,” he said. “It may be much more ubiquitous.”
The Power of Analogy
One area that’s seen some intriguing preliminary results is in social behavior. We think of society in spatial terms all the time: There are social ladders to climb, networks to build and expand, people we consider “close” or “distant.” Now, some research groups are probing social relationships for evidence of the grid code.
One recent study built up a two-dimensional space not unlike the bird experiment: People played a computer game, interacting with characters in ways that could change their levels of power or affiliation. The researchers found that the hippocampus seemed to track the positions of the characters in that space, relative to the test subject. Although the experiment did not determine whether the hippocampus is navigating that social information in a gridlike way, Matthew Schafer, a graduate student at the Icahn School of Medicine at Mount Sinai currently working on the project, expects to find the telltale hexadirectional signal. (He and others are now studying how that navigation might be disrupted or otherwise affected in people with conditions like autism spectrum disorder.)
These ideas could make it worthwhile to pursue clues hidden in other kinds of spatial metaphors, too: Neurons beyond place cells and grid cells, after all, might also have something to contribute. There are head direction cells that fire when an animal points its head in a particular direction, and speed cells that indicate the rate at which one moves through space, and even boundary cells that represent the location of walls or other environmental borders.
Studying these neurons in more abstract contexts might yield new insights. For instance, boundary cell activity has been reported for not just the borders of a physical space but also the borders between separate events in a temporal sequence. Could these neurons also play a role in forming borders between concepts, in creating distinct domains of knowledge in the brain? Or could head direction cells help one orient oneself within a given topic? The potential for such analogies is enormous.
The same goes for gaining a better understanding of diseases and other states. Wolber studies aging, and in one recently published paper, he and his colleagues examined how the spatial navigation grid code changes in elderly people. They found that the signal became less stable, with the grid fluctuating between orientations — and that people with less stable grids were also much less adept at keeping track of their relative location when blindfolded and led along a circuitous course. Wolbers suggests that if the grid code is used to process many kinds of information and memories, it’s possible that a pathology that destabilizes the spatial grid system might have a more general effect on the stability of memory and other areas of cognition.
Still, “at this stage,” he warned, “the available data is scarce. We have to be cautious.”
Kate Jeffery, a behavioral neuroscientist at University College London, agreed. Sure, the brain might use a common system to encode spatial and nonspatial knowledge, if the latter can be represented as varying continuously on a two-dimensional scale. But it’s also possible that some cognitive tasks are so complicated and unnatural that the brain is forced to rely on a spatial analog as a crutch to get through them. Perhaps the experiments on sound frequency and stretched birds tapped into this feature, Jeffery said.
A Unifying Framework
To further cement the grid code’s broader applications, then, researchers first hope to figure out how these cells may be working in more than two dimensions, given that higher-level knowledge tends to involve far more than pairs of qualities, like neck length and leg length, or power and association. This is something that’s currently being examined in flying bats, which navigate through three dimensions rather than just two.
Some researchers are making even bolder claims. Jeff Hawkins, the founder of the machine intelligence company Numenta, leads a team that’s working on applying the grid code not just to explain the memory-related functions of the hippocampal region but to understand the entire neocortex — and with it, to explain all of cognition, and how we model every aspect of the world around us. According to his “thousand brains theory of intelligence,” he said, “the cortex is not just processing sensory input alone, but rather processing and applying it to a location.” When he first thought of the idea, and how grid cells might be facilitating it, he added, “I jumped out of my chair, I was so excited.”
Imagine closing your eyes and wrapping your hands around an unidentified object: in this case, a coffee cup. Hawkins posits that the brain takes in information about the position of each patch of skin touching the cup’s surface, relative to the cup itself — much as the grid code allows you to know your body’s position in space, relative to the room you’re in. Each patch of skin generates an independent model of what it may be touching; all of those models then get cross-referenced to reach the conclusion that the object is indeed a coffee cup.
Hawkins thinks the same logic can apply to anything with a structured framework. “Everything we do — planning, mathematics, physics, language — would be based on the same principle,” he said. “I think we’re on a cusp here, where all of a sudden we’re going to have a new paradigm for understanding how the brain works.”
While the hypothesis has piqued interest among other researchers, they remain skeptical that grid cells will be found beyond the vicinity of the hippocampus, and say that Hawkins and his team have a long way to go to prove the power of their model.
Still, it provides a good starting point for thinking about how to improve artificial intelligence. If the grid framework is indeed a general one, it could be mimicked to build machines that are far more flexible, creative, general and powerful.
The field is just starting to grapple with these notions. For now, researchers are continuing to probe the activity of the hippocampus in a slew of different contexts, in hopes of finally uniting its memory and navigation functions once and for all. “When conceptual and cognitive ideas really start to connect with the very low-level neural data,” Stachenfeld said, “it’s really very satisfying.”
Jordana Cepelewicz is a staff writer at Quanta Magazine who covers biology.