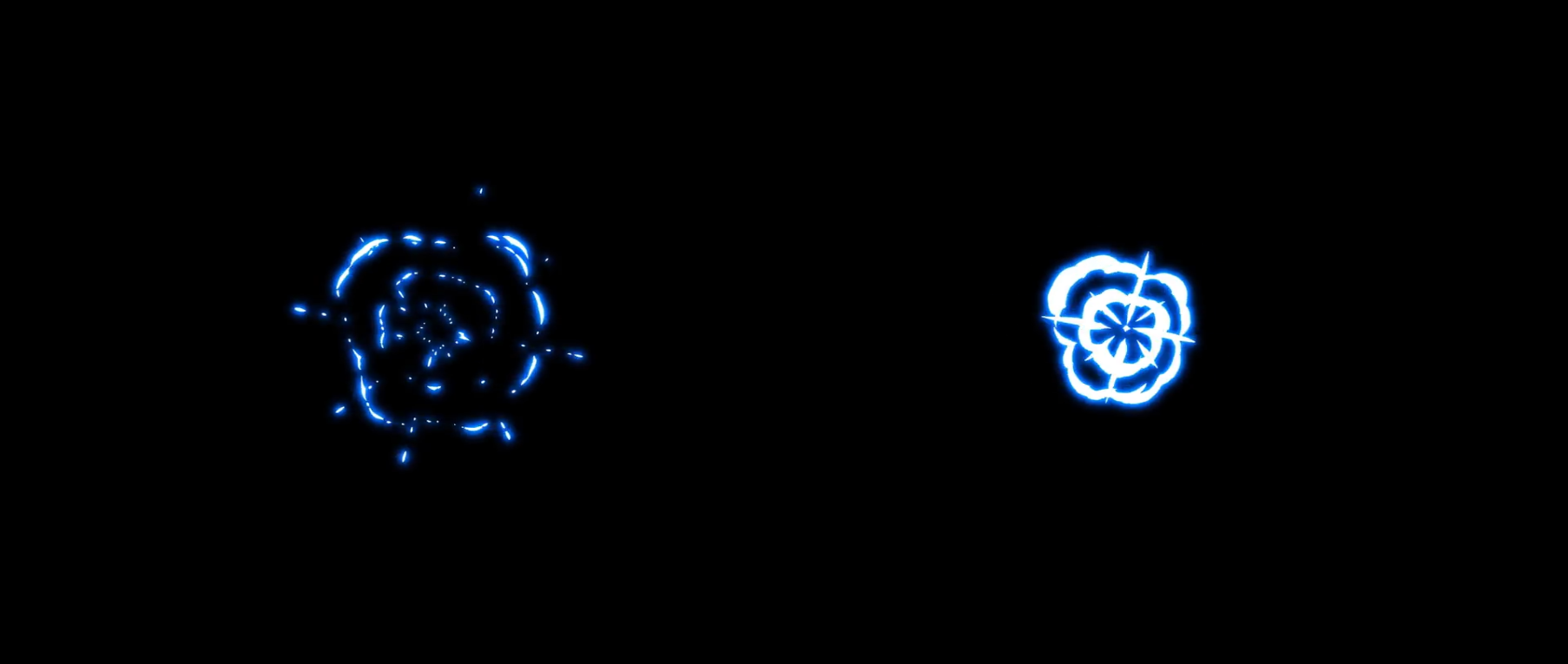
A quantum leap is a rapidly gradual process. Credit: Quanta Magazine; source: qoncha.
When quantum mechanics was first developed a century ago as a theory for understanding the atomic-scale world, one of its key concepts was so radical, bold and counter-intuitive that it passed into popular language: the “quantum leap.” Purists might object that the common habit of applying this term to a big change misses the point that jumps between two quantum states are typically tiny, which is precisely why they weren’t noticed sooner. But the real point is that they’re sudden. So sudden, in fact, that many of the pioneers of quantum mechanics assumed they were instantaneous.
A 2019 experiment shows that they aren’t. By making a kind of high-speed movie of a quantum leap, the work reveals that the process is as gradual as the melting of a snowman in the sun. “If we can measure a quantum jump fast and efficiently enough,” said Michel Devoret of Yale University, “it is actually a continuous process.” The study, which was led by Zlatko Minev, a graduate student in Devoret’s lab, was published on Monday in Nature. Already, colleagues are excited. “This is really a fantastic experiment,” said the physicist William Oliver of the Massachusetts Institute of Technology, who wasn’t involved in the work. “Really amazing.”
But there’s more. With their high-speed monitoring system, the researchers could spot when a quantum jump was about to appear, “catch” it halfway through, and reverse it, sending the system back to the state in which it started. In this way, what seemed to the quantum pioneers to be unavoidable randomness in the physical world is now shown to be amenable to control. We can take charge of the quantum.
All Too Random
The abruptness of quantum jumps was a central pillar of the way quantum theory was formulated by Niels Bohr, Werner Heisenberg and their colleagues in the mid-1920s, in a picture now commonly called the Copenhagen interpretation. Bohr had argued earlier that the energy states of electrons in atoms are “quantized”: Only certain energies are available to them, while all those in between are forbidden. He proposed that electrons change their energy by absorbing or emitting quantum particles of light — photons — that have energies matching the gap between permitted electron states. This explained why atoms and molecules absorb and emit very characteristic wavelengths of light — why many copper salts are blue, say, and sodium lamps yellow.
Bohr and Heisenberg began to develop a mathematical theory of these quantum phenomena in the 1920s. Heisenberg’s quantum mechanics enumerated all the allowed quantum states, and implicitly assumed that jumps between them are instant — discontinuous, as mathematicians would say. “The notion of instantaneous quantum jumps … became a foundational notion in the Copenhagen interpretation,” historian of science Mara Beller has written.
Another of the architects of quantum mechanics, the Austrian physicist Erwin Schrödinger, hated that idea. He devised what seemed at first to be an alternative to Heisenberg’s math of discrete quantum states and instant jumps between them. Schrödinger’s theory represented quantum particles in terms of wavelike entities called wave functions, which changed only smoothly and continuously over time, like gentle undulations on the open sea. Things in the real world don’t switch suddenly, in zero time, Schrödinger thought — discontinuous “quantum jumps” were just a figment of the mind. In a 1952 paper called “Are there quantum jumps?,” Schrödinger answered with a firm “no,” his irritation all too evident in the way he called them “quantum jerks.”
The argument wasn’t just about Schrödinger’s discomfort with sudden change. The problem with a quantum jump was also that it was said to just happen at a random moment — with nothing to say why that particular moment. It was thus an effect without a cause, an instance of apparent randomness inserted into the heart of nature. Schrödinger and his close friend Albert Einstein could not accept that chance and unpredictability reigned at the most fundamental level of reality. According to the German physicist Max Born, the whole controversy was therefore “not so much an internal matter of physics, as one of its relation to philosophy and human knowledge in general.” In other words, there’s a lot riding on the reality (or not) of quantum jumps.
Seeing Without Looking
To probe further, we need to see quantum jumps one at a time. In 1986, three teams of researchers reported them happening in individual atoms suspended in space by electromagnetic fields. The atoms flipped between a “bright” state, where they could emit a photon of light, and a “dark” state that did not emit at random moments, remaining in one state or the other for periods of between a few tenths of a second and a few seconds before jumping again. Since then, such jumps have been seen in various systems, ranging from photons switching between quantum states to atoms in solid materials jumping between quantized magnetic states. In 2007 a team in France reported jumps that correspond to what they called “the birth, life and death of individual photons.”
In these experiments the jumps indeed looked abrupt and random — there was no telling, as the quantum system was monitored, when they would happen, nor any detailed picture of what a jump looked like. The Yale team’s setup, by contrast, allowed them to anticipate when a jump was coming, then zoom in close to examine it. The key to the experiment is the ability to collect just about all of the available information about it, so that none leaks away into the environment before it can be measured. Only then can they follow single jumps in such detail.
The quantum systems the researchers used are much larger than atoms, consisting of wires made from a superconducting material — sometimes called “artificial atoms” because they have discrete quantum energy states analogous to the electron states in real atoms. Jumps between the energy states can be induced by absorbing or emitting a photon, just as they are for electrons in atoms.
Devoret and colleagues wanted to watch a single artificial atom jump between its lowest-energy (ground) state and an energetically excited state. But they couldn’t monitor that transition directly, because making a measurement on a quantum system destroys the coherence of the wave function — its smooth wavelike behavior — on which quantum behavior depends. To watch the quantum jump, the researchers had to retain this coherence. Otherwise they’d “collapse” the wave function, which would place the artificial atom in one state or the other. This is the problem famously exemplified by Schrödinger’s cat, which is allegedly placed in a coherent quantum “superposition” of live and dead states but becomes only one or the other when observed.
To get around this problem, Devoret and colleagues employ a clever trick involving a second excited state. The system can reach this second state from the ground state by absorbing a photon of a different energy. The researchers probe the system in a way that only ever tells them whether the system is in this second “bright” state, so named because it’s the one that can be seen. The state to and from which the researchers are actually looking for quantum jumps is, meanwhile, the “dark” state — because it remains hidden from direct view.
The researchers placed the superconducting circuit in an optical cavity (a chamber in which photons of the right wavelength can bounce around) so that, if the system is in the bright state, the way that light scatters in the cavity changes. Every time the bright state decays by emission of a photon, the detector gives off a signal akin to a Geiger counter’s “click.”
The key here, said Oliver, is that the measurement provides information about the state of the system without interrogating that state directly. In effect, it asks whether the system is in, or is not in, the ground and dark states collectively. That ambiguity is crucial for maintaining quantum coherence during a jump between these two states. In this respect, said Oliver, the scheme that the Yale team has used is closely related to those employed for error correction in quantum computers. There, too, it’s necessary to get information about quantum bits without destroying the coherence on which the quantum computation relies. Again, this is done by not looking directly at the quantum bit in question but probing an auxiliary state coupled to it.
The strategy reveals that quantum measurement is not about the physical perturbation induced by the probe but about what you know (and what you leave unknown) as a result. “Absence of an event can bring as much information as its presence,” said Devoret. He compares it to the Sherlock Holmes story in which the detective infers a vital clue from the “curious incident” in which a dog did not do anything in the night. Borrowing from a different (but often confused) dog-related Holmes story, Devoret calls it “Baskerville’s Hound meets Schrödinger’s Cat.”
To Catch a Jump
The Yale team saw a series of clicks from the detector, each signifying a decay of the bright state, arriving typically every few microseconds. This stream of clicks was interrupted approximately every few hundred microseconds, apparently at random, by a hiatus in which there were no clicks. Then after a period of typically 100 microseconds or so, the clicks resumed. During that silent time, the system had presumably undergone a transition to the dark state, since that’s the only thing that can prevent flipping back and forth between the ground and bright states.
So here in these switches from “click” to “no-click” states are the individual quantum jumps — just like those seen in the earlier experiments on trapped atoms and the like. However, in this case Devoret and colleagues could see something new.
Before each jump to the dark state, there would typically be a short spell where the clicks seemed suspended: a pause that acted as a harbinger of the impending jump. “As soon as the length of a no-click period significantly exceeds the typical time between two clicks, you have a pretty good warning that the jump is about to occur,” said Devoret.
That warning allowed the researchers to study the jump in greater detail. When they saw this brief pause, they switched off the input of photons driving the transitions. Surprisingly, the transition to the dark state still happened even without photons driving it — it is as if, by the time the brief pause sets in, the fate is already fixed. So although the jump itself comes at a random time, there is also something deterministic in its approach.
With the photons turned off, the researchers zoomed in on the jump with fine-grained time resolution to see it unfold. Does it happen instantaneously — the sudden quantum jump of Bohr and Heisenberg? Or does it happen smoothly, as Schrödinger insisted it must? And if so, how?
The team found that jumps are in fact gradual. That’s because, even though a direct observation could reveal the system only as being in one state or another, during a quantum jump the system is in a superposition, or mixture, of these two end states. As the jump progresses, a direct measurement would be increasingly likely to yield the final rather than the initial state. It’s a bit like the way our decisions may evolve over time. You can only either stay at a party or leave it — it’s a binary choice — but as the evening wears on and you get tired, the question “Are you staying or leaving?” becomes increasingly likely to get the answer “I’m leaving.”
The techniques developed by the Yale team reveal the changing mindset of a system during a quantum jump. Using a method called tomographic reconstruction, the researchers could figure out the relative weightings of the dark and ground states in the superposition. They saw these weights change gradually over a period of a few microseconds. That’s pretty fast, but it’s certainly not instantaneous.
What’s more, this electronic system is so fast that the researchers could “catch” the switch between the two states as it is happening, then reverse it by sending a pulse of photons into the cavity to boost the system back to the dark state. They can persuade the system to change its mind and stay at the party after all.
Flash of Insight
The experiment shows that quantum jumps “are indeed not instantaneous if we look closely enough,” said Oliver, “but are coherent processes”: real physical events that unfold over time.
The gradualness of the “jump” is just what is predicted by a form of quantum theory called quantum trajectories theory, which can describe individual events like this. “It is reassuring that the theory matches perfectly with what is seen” said David DiVincenzo, an expert in quantum information at Aachen University in Germany, “but it’s a subtle theory, and we are far from having gotten our heads completely around it.”
The possibility of predicting quantum jumps just before they occur, said Devoret, makes them somewhat like volcanic eruptions. Each eruption happens unpredictably, but some big ones can be anticipated by watching for the atypically quiet period that precedes them. “To the best of our knowledge, this precursory signal [to a quantum jump] has not been proposed or measured before,” he said.
Devoret said that an ability to spot precursors to quantum jumps might find applications in quantum sensing technologies. For example, “in atomic clock measurements, one wants to synchronize the clock to the transition frequency of an atom, which serves as a reference,” he said. But if you can detect right at the start if the transition is about to happen, rather than having to wait for it to be completed, the synchronization can be faster and therefore more precise in the long run.
DiVincenzo thinks that the work might also find applications in error correction for quantum computing, although he sees that as “quite far down the line.” To achieve the level of control needed for dealing with such errors, though, will require this kind of exhaustive harvesting of measurement data — rather like the data-intensive situation in particle physics, said DiVincenzo.
The real value of the result is not, though, in any practical benefits; it’s a matter of what we learn about the workings of the quantum world. Yes, it is shot through with randomness — but no, it is not punctuated by instantaneous jerks. Schrödinger, aptly enough, was both right and wrong at the same time.
Philip Ball is a science writer and author based in London who contributes frequently to Nature, New Scientist, Prospect, Nautilus and The Atlantic, among other publications.