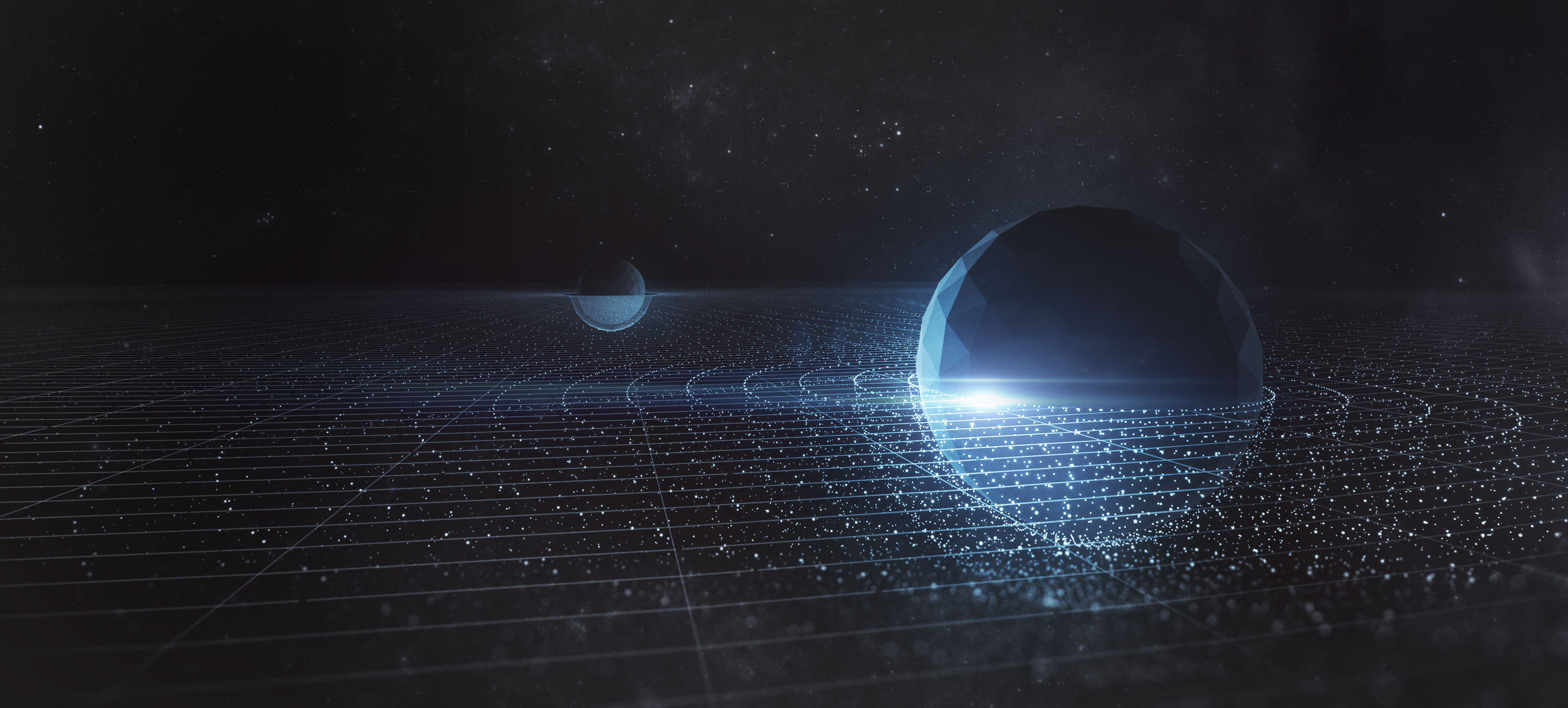
Two microdiamonds would be used to test the quantum nature of gravity. Credit: Olena Shmahalo / Quanta Magazine.
In 1935, when both quantum mechanics and Albert Einstein’s general theory of relativity were young, a little-known Soviet physicist named Matvei Bronstein, just 28 himself, made the first detailed study of the problem of reconciling the two in a quantum theory of gravity. This “possible theory of the world as a whole,” as Bronstein called it, would supplant Einstein’s classical description of gravity, which casts it as curves in the space-time continuum, and rewrite it in the same quantum language as the rest of physics.
Bronstein figured out how to describe gravity in terms of quantized particles, now called gravitons, but only when the force of gravity is weak — that is (in general relativity), when the space-time fabric is so weakly curved that it can be approximated as flat. When gravity is strong, “the situation is quite different,” he wrote. “Without a deep revision of classical notions, it seems hardly possible to extend the quantum theory of gravity also to this domain.”
His words were prophetic. Eighty-three years later, physicists are still trying to understand how space-time curvature emerges on macroscopic scales from a more fundamental, presumably quantum picture of gravity; it’s arguably the deepest question in physics. Perhaps, given the chance, the whip-smart Bronstein might have helped to speed things along. Aside from quantum gravity, he contributed to astrophysics and cosmology, semiconductor theory, and quantum electrodynamics, and he also wrote several science books for children, before being caught up in Stalin’s Great Purge and executed in 1938, at the age of 31.
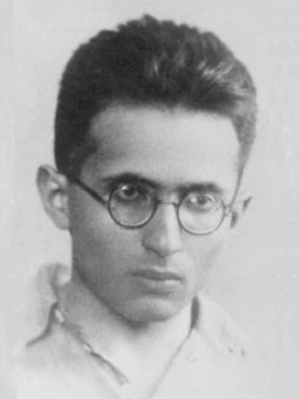
The Soviet theoretical physicist Matvei Petrovich Bronstein (1906-1938), a pioneer of quantum gravity research whose work remains largely unknown in the west. Photo from the Public Domain.
The search for the full theory of quantum gravity has been stymied by the fact that gravity’s quantum properties never seem to manifest in actual experience. Physicists never get to see how Einstein’s description of the smooth space-time continuum, or Bronstein’s quantum approximation of it when it’s weakly curved, goes wrong.
The problem is gravity’s extreme weakness. Whereas the quantized particles that convey the strong, weak and electromagnetic forces are so powerful that they tightly bind matter into atoms, and can be studied in tabletop experiments, gravitons are individually so weak that laboratories have no hope of detecting them. To detect a graviton with high probability, a particle detector would have to be so huge and massive that it would collapse into a black hole. This weakness is why it takes an astronomical accumulation of mass to gravitationally influence other massive bodies, and why we only see gravity writ large.
Not only that, but the universe appears to be governed by a kind of cosmic censorship: Regions of extreme gravity — where space-time curves so sharply that Einstein’s equations malfunction and the true, quantum nature of gravity and space-time must be revealed — always hide behind the horizons of black holes.
“Even a few years ago it was a generic consensus that, most likely, it’s not even conceivably possible to measure quantization of the gravitational field in any way,” said Igor Pikovski, a theoretical physicist at Harvard University.
Now, a pair of papers published in 2017 in Physical Review Letters has changed the calculus. The papers contend that it’s possible to access quantum gravity after all — while learning nothing about it. The papers, written by Sougato Bose at University College London and nine collaborators and by Chiara Marletto and Vlatko Vedral at the University of Oxford, propose a technically challenging, but feasible, tabletop experiment that could confirm that gravity is a quantum force like all the rest, without ever detecting a graviton. Miles Blencowe, a quantum physicist at Dartmouth College who was not involved in the work, said the experiment would detect a sure sign of otherwise invisible quantum gravity — the “grin of the Cheshire cat.”
The proposed experiment will determine whether two objects — Bose’s group plans to use a pair of microdiamonds — can become quantum-mechanically entangled with each other through their mutual gravitational attraction. Entanglement is a quantum phenomenon in which particles become inseparably entwined, sharing a single physical description that specifies their possible combined states. (The coexistence of different possible states, called a “superposition,” is the hallmark of quantum systems.) For example, an entangled pair of particles might exist in a superposition in which there’s a 50 percent chance that the “spin” of particle A points upward and B’s points downward, and a 50 percent chance of the reverse. There’s no telling in advance which outcome you’ll get when you measure the particles’ spin directions, but you can be sure they’ll point opposite ways.
The authors argue that the two objects in their proposed experiment can become entangled with each other in this way only if the force that acts between them — in this case, gravity — is a quantum interaction, mediated by gravitons that can maintain quantum superpositions. “If you can do the experiment and you get entanglement, then according to those papers, you have to conclude that gravity is quantized,” Blencowe explained.
To Entangle a Diamond
Quantum gravity is so imperceptible that some researchers have questioned whether it even exists. The venerable mathematical physicist Freeman Dyson, 94, has argued since 2001 that the universe might sustain a kind of “dualistic” description, where “the gravitational field described by Einstein’s theory of general relativity is a purely classical field without any quantum behavior,” as he wrote that year in The New York Review of Books, even though all the matter within this smooth space-time continuum is quantized into particles that obey probabilistic rules.
Dyson, who helped develop quantum electrodynamics (the theory of interactions between matter and light) and is professor emeritus at the Institute for Advanced Study in Princeton, New Jersey, where he overlapped with Einstein, disagrees with the argument that quantum gravity is needed to describe the unreachable interiors of black holes. And he wonders whether detecting the hypothetical graviton might be impossible, even in principle. In that case, he argues, quantum gravity is metaphysical, rather than physics.
He is not the only skeptic. The renowned British physicist Sir Roger Penrose and, independently, the Hungarian researcher Lajos Diósi have hypothesized that space-time cannot maintain superpositions. They argue that its smooth, solid, fundamentally classical nature prevents it from curving in two different possible ways at once — and that its rigidity is exactly what causes superpositions of quantum systems like electrons and photons to collapse. This “gravitational decoherence,” in their view, gives rise to the single, rock-solid, classical reality experienced at macroscopic scales.
The ability to detect the “grin” of quantum gravity would seem to refute Dyson’s argument. It would also kill the gravitational decoherence theory, by showing that gravity and space-time do maintain quantum superpositions.
Bose’s and Marletto’s proposals appeared simultaneously mostly by chance, though experts said they reflect the zeitgeist. Experimental quantum physics labs around the world are putting ever-larger microscopic objects into quantum superpositions and streamlining protocols for testing whether two quantum systems are entangled. The proposed experiment will have to combine these procedures while requiring further improvements in scale and sensitivity; it could take a decade or more to pull it off. “But there are no physical roadblocks,” said Pikovski, who also studies how laboratory experiments might probe gravitational phenomena. “I think it’s challenging, but I don’t think it’s impossible.”
The plan is laid out in greater detail in the paper by Bose and co-authors — an Ocean’s Eleven cast of experts for different steps of the proposal. In his lab at the University of Warwick, for instance, co-author Gavin Morley is working on step one, attempting to put a microdiamond in a quantum superposition of two locations. To do this, he’ll embed a nitrogen atom in the microdiamond, next to a vacancy in the diamond’s structure, and zap it with a microwave pulse. An electron orbiting the nitrogen-vacancy system both absorbs the light and doesn’t, and the system enters a quantum superposition of two spin directions — up and down — like a spinning top that has some probability of spinning clockwise and some chance of spinning counterclockwise. The microdiamond, laden with this superposed spin, is subjected to a magnetic field, which makes up-spins move left while down-spins go right. The diamond itself therefore splits into a superposition of two trajectories.
In the full experiment, the researchers must do all this to two diamonds — a blue one and a red one, say — suspended next to each other inside an ultracold vacuum. When the trap holding them is switched off, the two microdiamonds, each in a superposition of two locations, fall vertically through the vacuum. As they fall, the diamonds feel each other’s gravity. But how strong is their gravitational attraction?
If gravity is a quantum interaction, then the answer is: It depends. Each component of the blue diamond’s superposition will experience a stronger or weaker gravitational attraction to the red diamond, depending on whether the latter is in the branch of its superposition that’s closer or farther away. And the gravity felt by each component of the red diamond’s superposition similarly depends on where the blue diamond is.
In each case, the different degrees of gravitational attraction affect the evolving components of the diamonds’ superpositions. The two diamonds become interdependent, meaning that their states can only be specified in combination — if this, then that — so that, in the end, the spin directions of their two nitrogen-vacancy systems will be correlated.
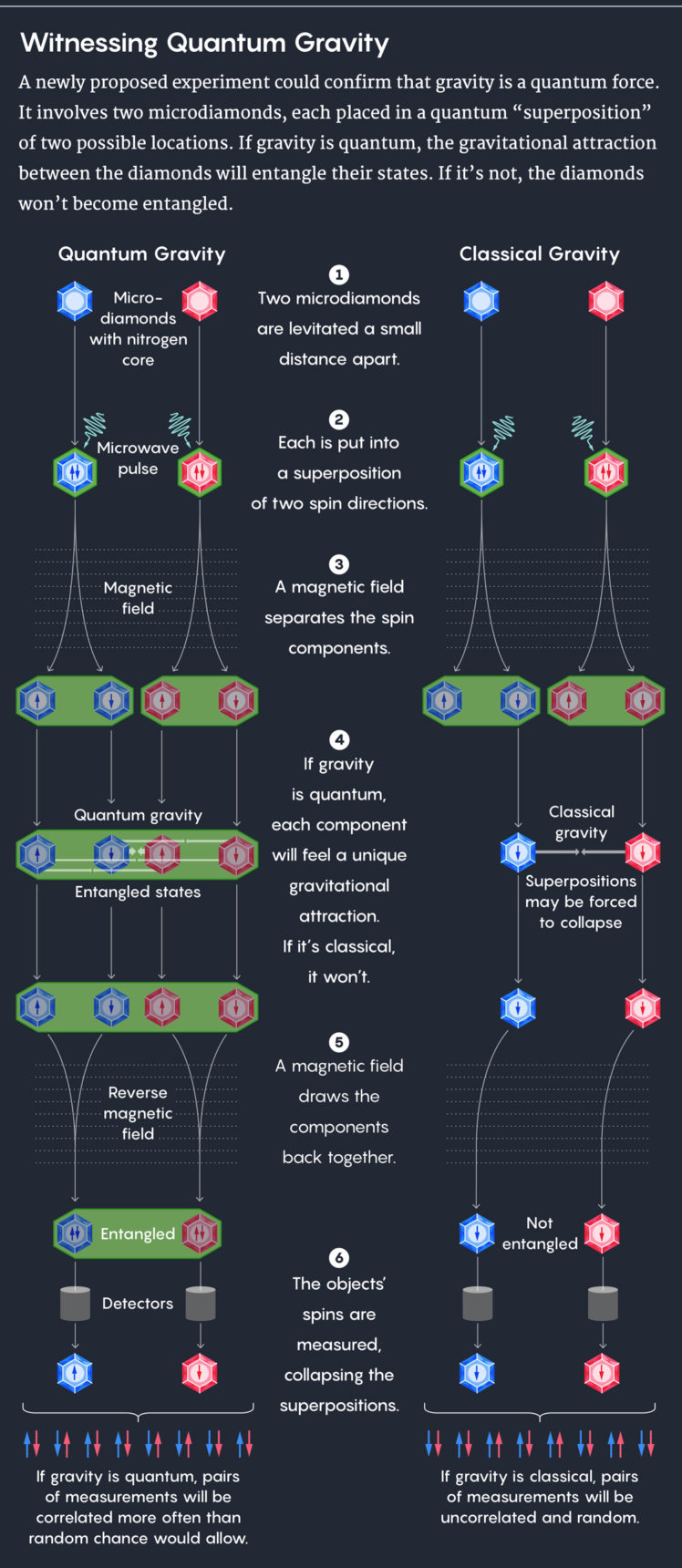
Credit: Lucy Reading-Ikkanda / Quanta Magazine.
After the microdiamonds have fallen side by side for about three seconds — enough time to become entangled by each other’s gravity — they then pass through another magnetic field that brings the branches of each superposition back together. The last step of the experiment is an “entanglement witness” protocol developed by the Dutch physicist Barbara Terhal and others: The blue and red diamonds enter separate devices that measure the spin directions of their nitrogen-vacancy systems. (Measurement causes superpositions to collapse into definite states.) The two outcomes are then compared. By running the whole experiment over and over and comparing many pairs of spin measurements, the researchers can determine whether the spins of the two quantum systems are correlated with each other more often than a known upper bound for objects that aren’t quantum-mechanically entangled. In that case, it would follow that gravity does entangle the diamonds and can sustain superpositions.
“What’s beautiful about the arguments is that you don’t really need to know what the quantum theory is, specifically,” Blencowe said. “All you have to say is there has to be some quantum aspect to this field that mediates the force between the two particles.”
Technical challenges abound. The largest object that’s been put in a superposition of two locations before is an 800-atom molecule. Each microdiamond contains more than 100 billion carbon atoms — enough to muster a sufficient gravitational force. Unearthing its quantum-mechanical character will require colder temperatures, a higher vacuum and finer control. “So much of the work is getting this initial superposition up and running,” said Peter Barker, a member of the experimental team based at UCL who is improving methods for laser-cooling and trapping the microdiamonds. If it can be done with one diamond, Bose added, “then two doesn’t make much of a difference.”
Why Gravity Is Unique
Quantum gravity researchers do not doubt that gravity is a quantum interaction, capable of inducing entanglement. Certainly, gravity is special in some ways, and there’s much to figure out about the origin of space and time, but quantum mechanics must be involved, they say. “It doesn’t really make much sense to try to have a theory in which the rest of physics is quantum and gravity is classical,” said Daniel Harlow, a quantum gravity researcher at the Massachusetts Institute of Technology. The theoretical arguments against mixed quantum-classical models are strong (though not conclusive).
On the other hand, theorists have been wrong before, Harlow noted: “So if you can check, why not? If that will shut up these people” — meaning people who question gravity’s quantumness — “that’s great.”
Dyson wrote in an email, after reading the PRL papers, “The proposed experiment is certainly of great interest and worth performing with real quantum systems.” However, he said the authors’ way of thinking about quantum fields differs from his. “It is not clear to me whether [the experiment] would settle the question whether quantum gravity exists,” he wrote. “The question that I have been asking, whether a single graviton is observable, is a different question and may turn out to have a different answer.”
In fact, the way Bose, Marletto and their co-authors think about quantized gravity derives from how Bronstein first conceived of it in 1935. (Dyson called Bronstein’s paper “a beautiful piece of work” that he had not seen before.) In particular, Bronstein showed that the weak gravity produced by a small mass can be approximated by Newton’s law of gravity. (This is the force that acts between the microdiamond superpositions.) According to Blencowe, weak quantized-gravity calculations haven’t been developed much, despite being arguably more physically relevant than the physics of black holes or the Big Bang. He hopes the new experimental proposal will spur theorists to find out whether there are any subtle corrections to the Newtonian approximation that future tabletop experiments might be able to probe.
Leonard Susskind, a prominent quantum gravity and string theorist at Stanford University, saw value in carrying out the proposed experiment because “it provides an observation of gravity in a new range of masses and distances.” But he and other researchers emphasized that microdiamonds cannot reveal anything about the full theory of quantum gravity or space-time. He and his colleagues want to understand what happens at the center of a black hole, and at the moment of the Big Bang.
Perhaps one clue as to why it is so much harder to quantize gravity than everything else is that other force fields in nature exhibit a feature called “locality”: The quantum particles in one region of the field (photons in the electromagnetic field, for instance) are “independent of the physical entities in some other region of space,” said Mark Van Raamsdonk, a quantum gravity theorist at the University of British Columbia. But “there’s at least a bunch of theoretical evidence that that’s not how gravity works.”
In the best toy models of quantum gravity (which have space-time geometries that are simpler than those of the real universe), it isn’t possible to assume that the bendy space-time fabric subdivides into independent 3-D pieces, Van Raamsdonk said. Instead, modern theory suggests that the underlying, fundamental constituents of space “are organized more in a 2-D way.” The space-time fabric might be like a hologram, or a video game: “Even though the picture is three-dimensional, the information is stored in some two-dimensional computer chip,” he said. In that case, the 3-D world is illusory in the sense that different parts of it aren’t all that independent. In the video-game analogy, a handful of bits stored in the 2-D chip might encode global features of the game’s universe.
The distinction matters when you try to construct a quantum theory of gravity. The usual approach to quantizing something is to identify its independent parts — particles, say — and then apply quantum mechanics to them. But if you don’t identify the correct constituents, you get the wrong equations. Directly quantizing 3-D space, as Bronstein did, works to some extent for weak gravity, but the method fails when space-time is highly curved.
Witnessing the “grin” of quantum gravity would help motivate these abstract lines of reasoning, some experts said. After all, even the most sensible theoretical arguments for the existence of quantum gravity lack the gravitas of experimental facts. When Van Raamsdonk explains his research in a colloquium or conversation, he said, he usually has to start by saying that gravity needs to be reconciled with quantum mechanics because the classical space-time description fails for black holes and the Big Bang, and in thought experiments about particles colliding at unreachably high energies. “But if you could just do this simple experiment and get the result that shows you that the gravitational field was actually in a superposition,” he said, then the reason the classical description falls short would be self-evident: “Because there’s this experiment that suggests gravity is quantum.”
Natalie Wolchover is a senior writer and editor at Quanta Magazine covering the physical sciences.